Research and Projects
Chemistry and Biochemistry
Dr. Thomas Arruda, associate professor

Energy is a major scientific, economic and societal challenge. Our current demand for fossil fuels is problematic for reasons that include environmental destruction and long-term supply inadequacies. Clean, renewable alternatives must be developed to mitigate these issues.
Our research involves fundamental and applied investigations into materials that are relevant to renewable energy alternatives, such as fuel cells and batteries. Fuel cells are electrochemical reactors that produce energy during chemical reactions, while batteries are storage facilities that can be used with other clean alternatives such as wind and solar.
With fuel cells, we primarily focus on the electrocatalysts that facilitate the chemical reactions that produce energy. For example, proton exchange membrane fuel cells require platinum catalysts that work well but limit their viability due to high cost. Cheaper alternatives will create a paradigm shift in power for electric vehicles and other industries. We are investigating alternatives to platinum for use in fuel cells, not only for vehicles but other applications such as space and technological applications.
With batteries, we focus on large-scale batteries that can leverage power to the grid during times of low output from renewable sources, or store energy when renewables are not needed by the grid. One variety is the redox-flow battery, which is similar to fuel cells but is usually simpler and does not require catalysts. We investigate factors that affect the performance of these batteries, such as flow dynamics and electrochemical kinetics.
We employ many techniques in our investigations, including electrochemical (cyclic voltammetry, chrono methods and electrochemical impedance spectroscopy) and spectroscopic (synchrotron based x-ray absorption and diffraction techniques). In addition to our in-house studies, we collaborate with colleagues at the Naval Undersea Warfare Center, Northeastern University, University of Dayton, Brookhaven National Lab and others.
Dr. Khadine Higgins, associate professor

We seek to understand how bacterial cells uptake, transport and export different transition metals. Our research utilizes a variety of chemistry, biochemistry and molecular biology techniques to study metal ion homeostasis in bacteria.
Our group is currently working on a transcriptional regulator from Mycobacterium tuberculosis (M. tuberculosis), KmtR, which is responsible for regulating the expression of an export protein for Ni(II) and Co(II). M. tuberculosis, the causative agent of tuberculosis, infects nearly one-third of the world’s population and is responsible for the death of almost 2 million people annually. The metal site structures of KmtR and the respective metal binding affinities are being determined.
Our long-term goal is to understand why M. tuberculosis requires two Ni(II)- and Co(II)-responsive metalloregulators. Metal ion homeostasis is critical for the survival of many organisms, and many pathogens require these essential metal ions for their survival. These metals can be toxic, and as a result, bacteria have developed ways of dealing with the changes in metal ion concentrations internally and within their environments. Understanding how pathogenic bacteria maintain intracellular concentrations of metal ions is of utmost importance, as the number of drug-resistant strains of bacteria continue to increase.
Dr. Susan Meschwitz, associate professor
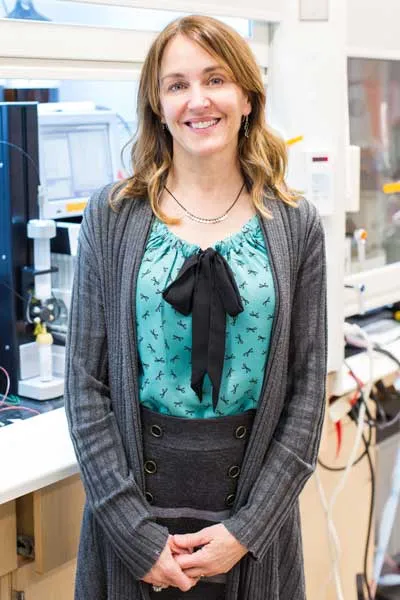
Our research lies at the interface of organic synthesis, medicinal chemistry and chemical biology. Our ultimate goal is to use chemical tools to solve problems of importance in biology and medicine.
We are interested in organic molecules that have the potential to impact modern medicine. The rise of drug-resistant bacteria that are extremely difficult to treat has emerged as a threat to public health. The failure of existing antibiotics to control infection makes it crucial to find alternatives to currently available drugs. Many pathogenic bacteria rely on a communication system known as quorum sensing to regulate virulence factors necessary for infection of a host.
Our long-term objective is to discover molecules capable of inhibiting quorum sensing. We are currently exploring two areas for the discovery of these compounds: design and synthesis in our laboratory and isolation from natural products. The molecules synthesized or isolated in our lab are anticipated to serve as valuable tools in the study of quorum sensing and to provide potential new leads in the development of anti-infective agents.
Dr. Bernard Munge, professor
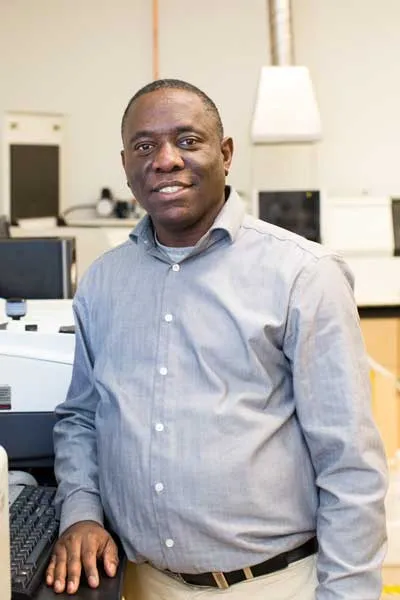
Our research focuses on the development of chemical and biological sensors. We span the traditional areas of bioanalytical chemistry, biology and material science, focusing on the development of nanomaterials-based sensor arrays for applications in biomedical diagnostics.
One ongoing project focuses on biosensor arrays for the early detection of cancer biomarker proteins in serum. Broadly defined as measurable or observable factors that indicate normal biological processes, disease processes or responses to a therapeutic intervention, biomarkers can include physical symptoms, secreted proteins, mutated DNAs and RNAs, cell death or proliferation, and serum concentrations of small molecules such as glucose or cholesterol.
The development of low-cost, reliable methods for simultaneously measuring panels of protein biomarkers is critically important for early detection of cancer, disease monitoring and personalized cancer therapy. Our long-term goal is to develop a simple, rapid device capable of simultaneously measuring a panel of 4-8 cancer biomarkers for point-of-care clinical application at the physician’s office. This will dramatically reduce health care costs and lead to accurate cancer diagnosis, disease monitoring and patients’ response to therapy and allow early detection of disease relapse.
We are also involved in statewide, collaborative research focused on developing micro-fluidic sensors with integrated nanoscale architectures and supramolecular chemistries for real-time, low-cost, rapid detection of nutrients and pollutants in ocean waters.
Students participating in our cancer biomarker research are exposed to many bioanalytical techniques that are applied to synthesize nanoparticles, fabricate the immunosensors, characterize and analyze the biosensor chemistry. These include voltammetry, UV-Vis spectroscopy, surface plasmon resonance, electrochemiluminescence, screen printing (fabrication), quartz crystal microbalance, spectroelectrochemistry, Fourier-transform infrared spectroscopy, Raman spectroscopy and surface chemistry techniques.
These research experiences are leading to unique career opportunities for our students. Our students are not only learning science but also doing science.